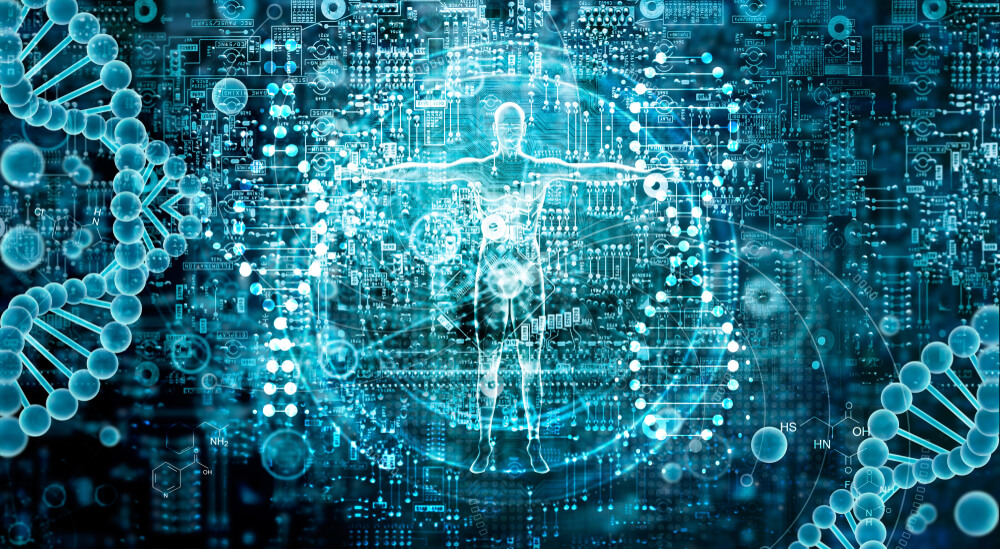
2021 will be a big year for emerging oncology therapeutics, as we anticipate major late-stage clinical advancements for several new modalities either reaching their first regulatory decision (e.g., TILs, TCR-T, first solid tumor bispecific antibody) or making the leap from newly emerging into more established with new targets or indications beyond their initial approvals (e.g., BCMA CAR-T in multiple myeloma, dual targeting bispecific antibodies in solid tumors). For more details on near-term timelines for specific emerging and established therapies, please see our recently published 2021 Oncology Outlook white paper here.
In this piece, we will introduce a high-level framework for considering the novelty of emerging modalities in oncology, with an overview of the underlying science and technology along with some clinical or near-clinical examples.
When we consider the novelty of a new or emerging therapeutic or class of therapeutics, we look at several dimensions:
Modality can be defined in different ways, but it typically refers to the general category of therapeutic design that accounts for the type of building block it uses and / or its general mechanism of action (e.g., monoclonal antibodies, small molecule inhibitors). At a high level, modalities can be categorized as follows:
- Small Molecule / Inorganic Material: intended to deliver therapeutic or directly disrupt or kill cancer cells (e.g., chemotherapy, Tyrosine Kinase Inhibitor (TKI), lipid nanoparticle):
- Nucleotides (DNA or RNA): intended to either express a cancer-fighting protein (DNA or mRNA) or suppress expression of a critical tumor cell gene (silencing RNAs)
- Peptides (protein or antibody): intended to bind a tumor-associated antigen to either directly block its function or to target cell-killing therapeutics to cancer cells
- Living therapeutic: a modified living cell designed to directly kill tumor cells or initiate a tumor-killing immune response (e.g., cell therapies, oncolytic virus)
Each type of modality has intrinsic properties that impact its bioavailability, efficacy, safety, ease of manufacturing, dosing, etc. It can be tempting to focus on targets as the main criteria for novelty, but because the modality is such a key driver of product attributes, a unique therapeutic design that modulates a known target can still be a novel therapeutic if it is first-in-class.
Given these considerations, classifying a specific therapy as novel is not always straightforward. We first consider a spectrum of established to emerging or more speculative modalities for oncology therapeutics. Some modalities like chemotherapy or small molecule TKIs are clearly well-established with multiple variations across a breadth of indications. Anything novel here would have to involve a completely new target or a major advancement in design or delivery.
Conversely, for some early stage or pre-clinical modalities like NK cell therapies or siRNA-based therapies, we can feel confident classifying them as emerging. However, there are several classes of therapy in which at least one therapeutic has been approved, but the class hasn’t quite “broken through” with additional targets or beyond the initial indication. These include CAR-Ts, bispecific T Cell Engagers (TCE), oncolytic viruses, and Dendritic Cell (DC) vaccines. These therapies we will consider as late-emerging (and still novel) for the purposes of this discussion, even though they have at least one approval.
Keeping this framework in mind, let’s review some recent exciting scientific and clinical breakthroughs in modality, target, and design:
Bispecific antibodies: better targeting and modular potential
The first (and only) marketed bispecific antibody (bsAb), Amgen’s CD19:CD3 T Cell Engager (TCE) BLINCYTO, was approved in r/r B-ALL just over 7 years ago. It has remained alone on the market since then.
Within liquid tumors, there are other TCE therapies approaching registrational stages, particularly the CD20:CD3 TCEs. Surprisingly, the next TCE regulatory decision is likely to be Immunocore’s gp100-specific-TCR:CD3 bispecific T Cell Engager, tebentafusp, in a solid tumor (uveal melanoma with a breakthrough designation and a planned FDA filing in 3Q21). This would be an important proof of concept for this modality in the solid tumor setting.
Even more surprising, the next bsAb approval may not be a TCE at all, but instead one or more non-T cell dual-targeting bsAbs in solid tumors, namely:
- J&J’s EGFR exon 20:MET bsAb amivantamab in NSCLC (submitted for FDA approval in Dec 2020)
- Zymework’s HER2:HER2 bsAb zanidatamab in HER2-amplified biliary cancer (FDA breakthrough designation granted Nov 2020).
These modular approaches show enormous potential for fine-tuning targeting strategies, as it enables more precise selection of tumor cells while better sparing healthy cells.
Further on the horizon, we see emerging pre-clinical designs and data for trispecific antibodies. For example, Sanofi’s CD38xCD28:CD3 trispecific T Cell Engager SAR442257, which is designed to direct T cells to multiple myeloma cells and boost their efficacy via CD28 stimulation. Taking it a step even further, researchers at Washington University recently published in vitro proof of concept data on NanoMuTes (nanoparticle multitarget T Cell engagers), which targets three separate targets toward a CD3 engager1.
Complementing and enhancing these modular designs, we are also seeing increasingly precise antibody design, targeted not just to proteins highly overexpressed in tumors and mostly absent from healthy cells (e.g., HER2), but more subtle misregulations like tumor-specific mutations or low level overexpression. For example, Johns Hopkins researchers have designed a p53:CD3 T Cell Engager that only targets mutated p53 (not healthy cells expressing normal p53)2.
Researchers are also developing bsAbs that are very sensitive to slightly overexpressed tumor proteins like RAS3. This will further support better targeting as these improved antibodies are mix-and-matched into various conjugated antibody designs. This will go beyond just bispecific and trispecifics to also include antibody-drug conjugates (ADCs), which use the antibody to direct a cytotoxic payload to the tumor cell.
RNA and Gene therapy: fine-tuning gene expression
For decades, targeted cancer therapeutics have been exclusively focused on misregulated cancer proteins (either overexpressed, or mutated) using small molecule and antibody-based approaches to block their oncogenic activity. But recently we have been seeing a prominent shift towards targeting the root cause for certain misregulations, namely modulating gene expression levels more directly with DNA and RNA-based therapeutics.
Unlike antibodies and small molecules, which almost always are used to block or suppress an overactive or overexpressed oncogenic protein’s function, DNA and mRNA-based therapies can induce protein expression. This unlocks an underdeveloped but rich arena of targets, the so-called “tumor suppressor” genes which are suppressed in tumor cells, but whose induced expression kills tumor cells. The most famous of these targets is p53, which has been targeted for decades now in hopes of leveraging its tumor-killing function. Another set of inducible targets is the immune stimulators, which have been targeted using monoclonal antibodies without much clinical success (e.g., OX40 agonist antibodies intended to activate this immune booster).
Conversely, other forms of silencing RNA such as siRNA or RNAi can be used to selectively down-repress gene expression, therefore targeting overexpressed oncogenic proteins or immune suppression at the source. For example, IMVAX’s IMV-001 protocol collects tumor cells, treats them with antisense IGF-1R to stress them, then implants them into the abdomen to trigger an immune cascade that targets the cancer cells.
The challenge with DNA and RNA-based therapies has long been ensuring a successful delivery into the tumor cell, because these nucleotides are almost immediately degraded when released “naked” into the body. Here, cancer therapeutics have built on the foundation of rare diseases by bringing gene therapy viral vector delivery approaches into the clinic. For example, Ziopharm’s “Controlled IL-12” (Ad-RTS-hIL-12 plus veledimex) is an adenovirus vector expressing the immune stimulator IL-12, along with an “on switch” that can be triggered inside glioblastoma cells by oral veledimex treatment.
Another interesting delivery approach is demonstrated by Genprex’s clinical stage Reqorsa, which is a single gene’s worth of DNA packaged into a lipid nanoparticle which stabilizes it and promotes cellular uptake. Once inside a cell, the gene TUCS2 is expressed. In NSCLC cells it restores normal cellular functions that stop growth and induce tumor cell death.
There is also foundational work being done on how to synthesize RNA to make it more stable while still maintaining its regulatory function. For example, Orna is working on a type of RNA called oRNA which is circularized (similar to a DNA plasmid) and can then exist longer within the cell4.
In addition to these clever delivery and stabilization methods, we also see an intriguing DNA/protein blended approach with the pre-clinical “vectorized antibodies”, which are DNA vectors that can express a disease-fighting antibody from inside a cell. Currently these are primarily being explored in rare diseases, but these could have major cancer therapeutic potential as well.
Another goal of gene expression can be to express tumor-specific antigens within the body in order to induce an immune response. Famously, this successful approach was used for the first approved COVID-19 vaccines, Pfizer’s and Moderna’s mRNA-based vaccines. Moderna and others are also pursuing this approach in oncology, with some early signs of potential efficacy.
Taken together, we are poised for dramatic advancement across multiple gene expression-focused modalities.
Living Therapies: making the leap from emerging to established
Given the complexity of fighting cancer without harming the patient, living therapeutics are uniquely suited to selectively identify and kill cancer cells without harming the rest of the body. Within the last 10 years, two broad classes of living therapeutics have begun to emerge. The first is the oncolytic viruses, which are genetically modified viruses designed to attack and kill tumor cells. Amgen’s IMLYGIC (also known as TVEC) is a herpes virus modified to selectively replicate in cancer cells only. It was FDA-approved in melanoma in 2005. As there have been no FDA approvals for oncolytic viruses since then, we still consider this class to be an emerging therapy. More recently, there have been promising clinical data reported for oncolytic viruses including Istari’s PVRIPO in glioblastoma – perhaps we will see the next breakthrough in this modality soon.
The other broad group of living therapies are cell-based therapies (see our in-depth report here). T cell therapies are the most advanced class within this group with some FDA approvals (CD19 CAR-T) and some near-term regulatory decisions (Iovance’s Tumor Infiltrating Lymphocyte / TIL lifoleucil in melanoma is already filed, and Adaptimmune’s SPEAR TCR-T cell platform potentially filing in 2022). Further ahead, we also anticipate potential breakthroughs for NK cell therapies as well as possibly DC vaccines. The latter, similar to oncolytic viruses, had a first approval some time ago with Dendreon’s PROVENGE in prostate cancer in 2010, but nothing since then.
Cell-based therapies, while very efficacious within their limited indications, are also very complex and expensive to produce, so innovations have also understandably been focused on manufacturing. Numerous developers are working on allogeneic (donor-derived or cell culture-derived) sources for these therapies to replace the current autologous model, which requires cells to be collected from each patient, modified, and then infused back. In parallel, many companies are working to replace the existing viral vector-based gene modifications with the more precise and scalable enzymatic approaches, e.g., CRISPR. Either—or both—of these shifts will dramatically boost the potential of cell-based therapies to reach broader success.
Looking further ahead, the vectorized protein delivery approach discussed above is also being explored in the context of CAR-T by Ensoma, where the goal would be to deliver TCR modifying gene vectors into the T cells, therefore inducing them to transform into CAR-T cells inside the body. This would completely bypass the current manufacturing process where cells are modified and maintained outside the body before use and could therefore be ground-breaking.
The Next Horizon: the machine revolution
As we continue to see advances within the established modalities of small molecule, protein, nucleotide, and living cell-based platforms, in parallel we are seeing increased focus on more synthetic approaches to fight cancer.
When we say synthetic, we include computationally-driven design. The first cancer genome was sequenced merely 15 years ago, but since then there has been an explosion of data on how cancer cell gene and protein expression is perturbed, along with increasingly advanced methods to understand and apply these learnings to the clinic. The most famous example of so-called “synthetic lethality” (where a particular target or combination is applied in a specific cancer genomic background in order to bypass resistance pathways) is the use of PARP inhibitors in BRCA-mutated tumors. Synthetic lethality can also include combinatorial approaches designed to knock out multiple players in the same pathways, for example using BRAF and MEK inhibitors in BRAF-mutated melanomas or combining CD19 mAbs and CD20:CD3 TCE in liquid cancers.
Over the next few years, we expect to do more than leverage synthetic approaches to improve design and targeting across modalities to better address some intractable protein targets (e.g., RAS, TP53, MET). We also expect more finely-tuned and personalized approaches based on individual tumor genomics. Ziopharm’s personalized TCR-T provides an example. It is a T cell therapy uniquely designed to each patient’s tumor genome. These approaches are not limited to clinical trials either, as more and more tumors are genomically-sequenced, individual physicians and some companies are exploring personalized cocktail and drug repurposing beyond approved labels.
Even more future-facing, researchers and developers are exploring the use of microscopic robots (nanomachines) in oncology. Bionaut Labs is developing nanobots that can circulate through the body and then drill into target tissues—like the brain—to deliver chemotherapies or other payloads directly into hard-to-access tumors like glioblastomas.
In conclusion, there is much to be optimistic about as we look to the future of oncology therapeutics. These and additional novel therapeutics should continue to deliver in both the lab and ultimately the clinic.